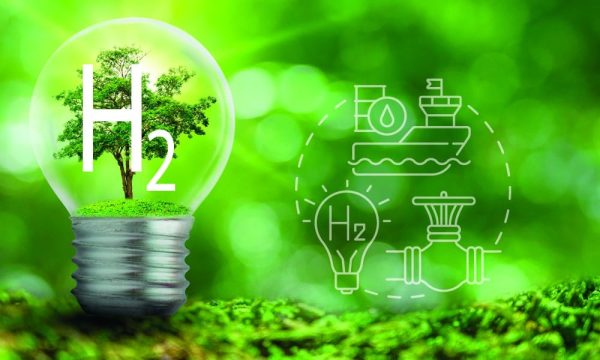
Hydrogen Valves and Typical Application Challenges
Modern hydrogen applications demand durable valves. Beside the challenges with the media hydrogen they also often have to operate reliably under high pressure or at extremely low temperatures. There are some challenges that the industries have to face. In this blog article, we describe relevant key data on hydrogen, hydrogen applications and hydrogen valves.
- petroleum refining
- glass purification
- semiconductor manufacturing
- fertilizer production
Other uses of hydrogen gas include hydrogenation of unsaturated fatty acids, heat-treating metals, pharmaceutical production and as a coolant in power plant generators.
These hydrogen applications have constituted a stable but limited market for the valve industry for many years.
In modern applications, hydrogen is increasingly being used as an energy carrier for the storage and delivery of large amounts of energy. Use of hydrogen as an energy carrier is not completely new. The first fuel cell was presented by Schönbein/Grove around 1838/39.
In the aerospace industry, NASA launched numerous space rockets using hydrogen as fuel during its Apollo space program which ran from 1961 to 1972.
Even though containers, pipes and valves for hydrogen use have existed for a long time, modern applications place higher demands on the performance of these components under more extreme conditions. Some of these applications require gas storage at high pressure and at extremely low temperatures.
The extreme conditions demand specially designed valves that can perform reliably under these conditions. Hydrogen presents additional challenges for plant engineering. Its small molecular size gives it high mobility.
What is hydrogen?
Hydrogen is the simplest and most abundant element on earth. It typically exists as a diatomic molecule (H2). At standard temperature and pressure, it is nontoxic, odorless, colorless, but a highly combustible gas.
How is hydrogen made?
Hydrogen is produced commercially by four main processes:
- Reformation: In the reformation process, methane is reacted with steam under pressure, in the presence of a catalyst, to produce hydrogen, carbon monoxide, and some carbon dioxide. E.g. 95% of the hydrogen produced in the United States is currently made by natural gas reforming.
- Electrolysis: In the electrolysis process electricity is used to split water into hydrogen and oxygen. This reaction takes place in a unit called an electrolyzer.
- Chlor-alkali production: The Chlor-alkali process produces hydrogen as a by-product along with chlorine and sodium hydroxide via the electrolysis of aqueous sodium chloride.
- Pyrolysis: Thermal decomposition of methane produces hydrogen and solid carbon. This method prevents the release of greenhouse gases during production as it yields solid carbon and no CO2.
Hydrogen is classified as green, turquoise, blue or gray depending on the production method and the amount of CO2 released into the atmosphere during the process.
Green hydrogen is produced through electrolysis using green electricity and water. It is virtually CO2 free and ideal for decarbonization of the economy.
Turquoise hydrogen is produced through pyrolysis of methane. This method generally produces solid carbon but CO2 is produced in the high temperature process.
Blue hydrogen is produced through the reformation process; low CO2 emissions are captured and stored instead of release into the atmosphere.
Gray hydrogen is produced through the electrolysis or reformation process in which CO2 is released into the atmosphere at the electricity generation or reformation stage.
For the achievement of climate goals through decarbonization, green hydrogen is the most useful, climate-neutral method of hydrogen production.
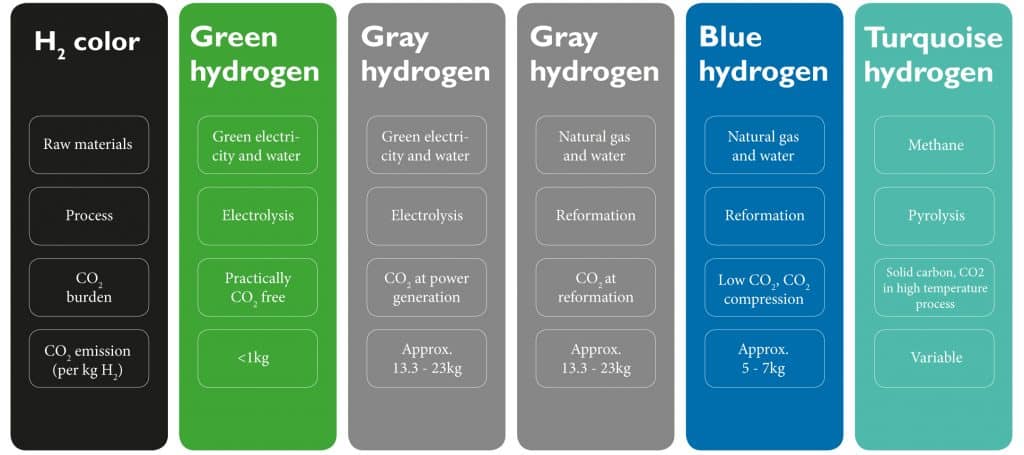
Colors of hydrogen (source: www.klimaschutz-niedersachsen.de and www.klimaandmore.de)
What is hydrogen used for?
Hydrogen is used in the industry for refining petroleum, treating metals, producing fertilizer, food processing and lowering the sulfur content of fuels. It is also used for clean energy generation. The figure below shows the complete cycle of hydrogen production and use.
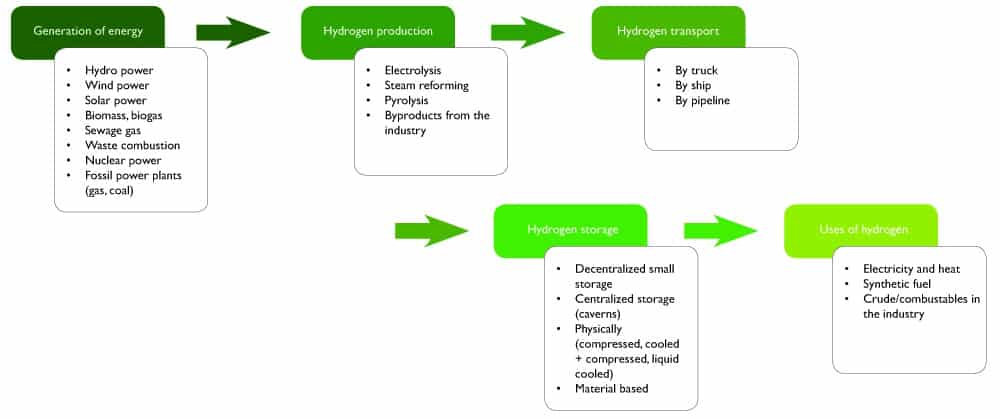
Hydrogen cycle from energy generation for production to gas use (source: AS-Schneider).
Energy generated from various sources is used for the production of hydrogen. The hydrogen produced is transported by various means to a centralized or decentralized storage where it is stored until use. The requirements for safely handling hydrogen at each stage of the cycle can differ significantly since the applications are as diverse and colorful as the spectrum of hydrogen. A single hydrogen valve that satisfies requirements for all processes does not exist. Different types of valves are suitable for different applications depending on the temperature and pressure requirements.
Sample application: Hydrogen as a fuel
Hydrogen has high energy content per unit mass which is nearly three times that of gasoline. The energy density per unit volume, however, is quite low at standard temperature and pressure. The volumetric energy density can be increased by storing the gas under higher pressure or in a liquified state at extremely low temperatures. In the field of commercial vehicle refueling, four different approaches are currently being considered and tested:
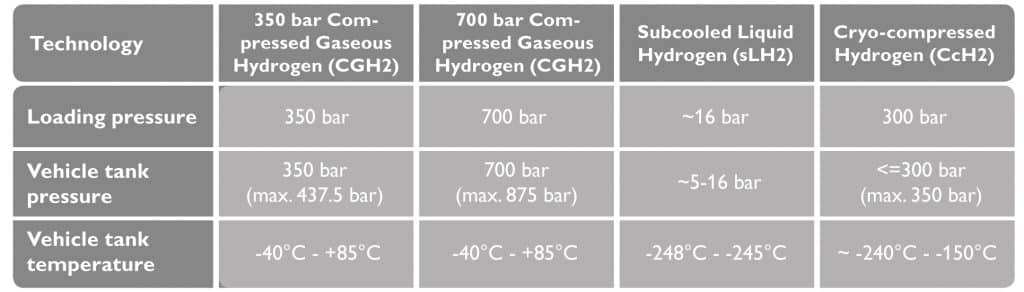
The use of hydrogen gas in commercial vehicle refueling (source: H2-Mobility).
The diverse temperature and pressure requirements present great challenges. But it offers big opportunities for the valve industry, too.
Hydrogen safety standards
The standardization around hydrogen as an energy storage medium is currently lagging behind the high dynamics of the market. Although various working groups have been formed to formalize and document the new requirements. The finalized and approved standards may still be months or even years away.
In view of the strong demand, both users and suppliers are currently looking for appropriate guidelines. One way to address this is to combine the various existing directives, codes and standards into a de facto standard until hydrogen regulations are available. The following existing guidelines, codes, and standards can be considered:
- Directives (e.g. pressure equipment directive)
- Standards and codes (ASME, ISO / TC, ISO / TR, SAE, etc.)
- Databases (e.g. from Sandia National Laboratories)
- Expertise from material testing institutes, universities, etc.
This can be provided by the supplier and used as a template for the customer agreement.
Typical application challenges for hydrogen service valves
The selection of a suitable valve for a given hydrogen application depends foremost on the functional requirements such as valve type, passage, connection type etc. In addition, the following type-specific aspects of the valve must also be carefully considered:
#1 Valve material for hydrogen service
Tiny hydrogen atoms can penetrate the crystal structure of solid metal and remain there. This effect leads to increased pressure and internal stresses without any corresponding increase in the strength of the material.
Hydrogen absorption can make the metal walls brittle, which may lead to crack formation and eventual failure.
Furthermore, the tensile strength, toughness and ductility of the metal are reduced that can result in violating the elongation-at-break requirement specified in the European Pressure Equipment Directive 2014/68 / EU.
Use of special high-strength materials that often work in other challenging situations, unfortunately does not solve this problem.
On the contrary, these materials are even more vulnerable to hydrogen embrittlement. The lowest sensitivity to hydrogen embrittlement is found in face-centered cubic metal grids such as those in austenitic steel (e.g. 316 / 316L) or aluminum.
But even with these materials, it is still important to avoid other pitfalls. In the case of 316 / 316L, for example, the hydrogen compatibility depends on the nickel content. This dependency is most pronounced between room temperature and approximately -40°C. To account for this effect, a steel with appropriate nickel content must be selected (if available on the market). The stress level may also be lowered through a reduced utilization of the component as done also with unalloyed steels.
It is also important to ensure that non-metallic valve materials such as seals and lubricants are compatible with hydrogen. A list of recommendations can be found in the standards or databases mentioned above (Sandia, ISO / TR, SAE).
#2 Valve production for hydrogen service
In addition to careful selection of suitable materials, it is important to ensure that further processing of these materials does not compromise their integrity. For example, the welding process can produce notches and residual stresses from local plasticization, which may then promote hydrogen embrittlement. Follow-up treatment according to NACE is recommended in these cases.
#3 Leakage-free hydrogen application
Since hydrogen is the smallest and lightest molecule, it has the ability to crawl through very small grooves and cracks causing leakage. Very small leaks in an open environment may not be dangerous.
However, in a closed space such as a protective box, even small leaks can produce a flammable mixture. It is therefore very important to carefully examine the qualification and accompanying test results of hydrogen valves.
For leakage testing, the use of inert-gas according to DIN EN ISO 14175 is normally recommended (e.g. 95/5 – 95% nitrogen with 5% hydrogen).
However, it is challenging to create a stable test environment for repeated measurements during continuous production. Battery charging, welding works or even normal breathing may cause the concentration of hydrogen in the environment to fluctuate influencing the measurements.
For this reason, measurement experts recommend use of helium as a test medium for hydrogen valves. Helium testing produces more representative results as the ambient conditions are much more stable with respect to the inert gas.
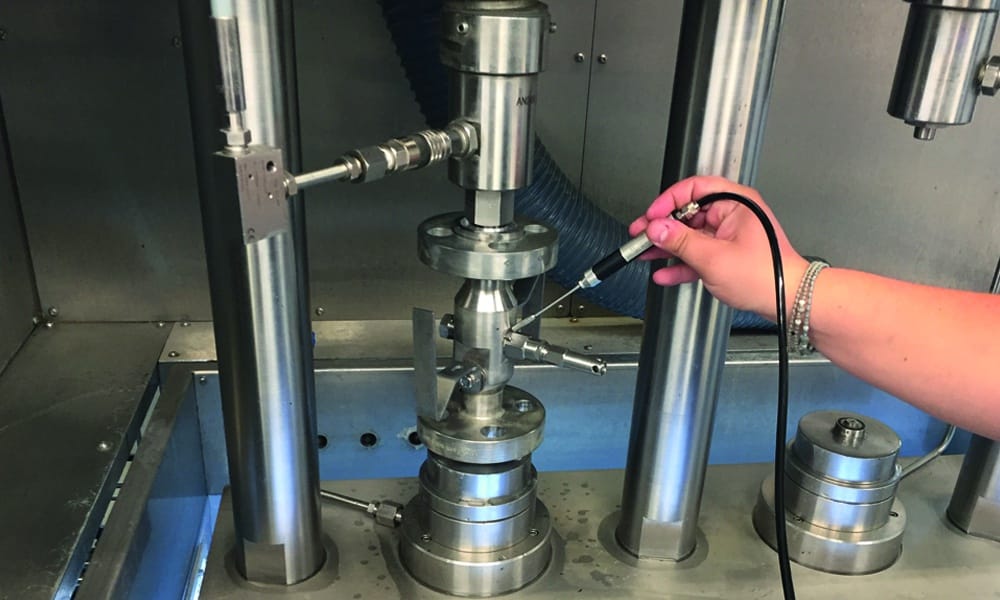
Carrying out a leak test using helium.
Summary
The hopes of achieving a net carbon-neutral economy during the next three decades rest heavily on the production and use of green hydrogen.
Hydrogen valves are key components in the safe production, storage, transportation and use of hydrogen.
The extremely flammable nature of hydrogen coupled with its ability to embrittle metals presents significant challenges in valve design and construction.
Metals and non-metallic materials used in the construction of hydrogen valves must be compatible with hydrogen. It must also be ensured that further processing of these materials does not make them vulnerable to hydrogen embrittlement.
Finally, it is essential that valves be suitably tested to ensure that they are leakage free.
If valves for hydrogen service are selected with these considerations in mind, they can be safely operated for an extended period of time.
Standardization of hydrogen valves is currently in progress. Until a unified final standard emerges, it is essential that plant engineers work in close coordination with suppliers for valve selection, qualification, and validation of existing installations. Material testing institutes at local universities can also be helpful for consultation and equipment testing.
The use of hydrogen as an energy carrier is an important part of a reduced CO2 future. Availability of safe and suitable hydrogen service valves is not an obstacle in the realization of this goal.
Via AS Schneider